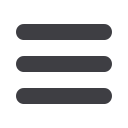
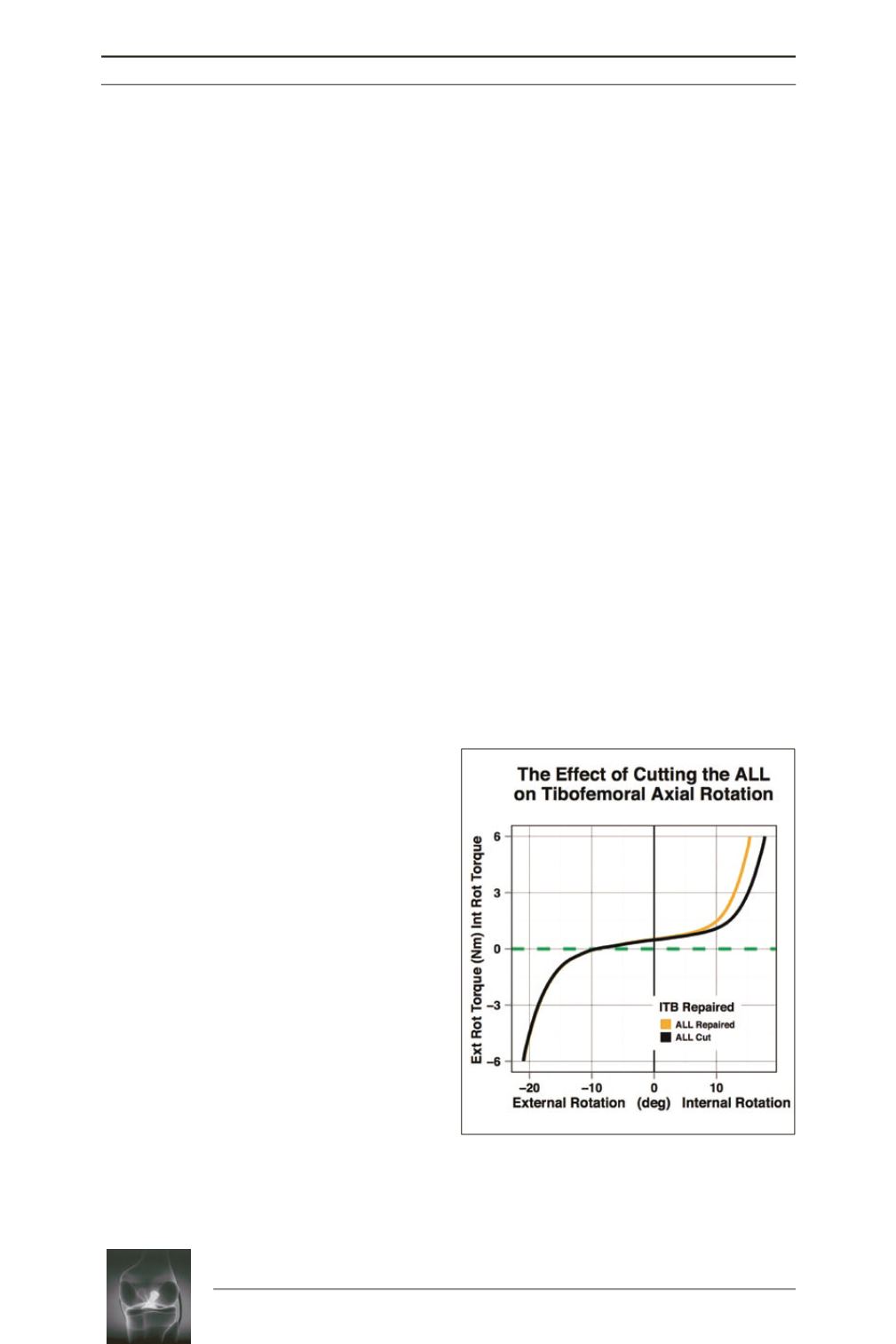
T. LORDING, A. GETGOOD, T.P. BRANCH
32
with anatomical and histological studies [20-
24], and descriptions of radiographic landmarks
[25, 26]. While the tibial insertion appears
relatively constant in these descriptions,
variation has been reported in the femoral
attachment. Some authors have described this
origin as being proximal and posterior to the
LCL [22-24], while others have described an
anterior and distal origin [19, 20]. Caterine
identified both variants, and also identified a
peripheral nervous innervation, suggesting a
role in proprioception [21].
Kennedy investigated the biomechanical
properties and failure mechanisms of the ALL,
finding a mean maximum load of 175 N and
stiffness of 20 N/mm [23]. In 12 specimens,
four mechanisms of failure were identified;
ligamentous tear at the femoral attachment in
four specimens, at the tibial insertion in one, in
the mid substance in four, and by a bony
avulsion (Ségond fracture) in six, although it
should be noted that the line of pull in these
experiments was non-physiologic. Dodds
determined the ligament to be isometric from
0-60° of flexion, and to lengthen with internal
tibial rotation, suggesting a role in internal
rotational control [22]. Kittl studied the
isometry of the native anterolateral structures
as well as potential points for the fixation of an
extra-articular reconstructions [27]. He found
an ALL with an origin posterior and proximal
to the LCL to be relatively isometric, whilst an
ALL with a distal and anterior origin was lax
approaching extension and unlikely to be
effective in controlling the pivot shift.
A number of authors have now investigated the
role of the ALL in rotational control of the
knee, with conflicting results. Lording and
Branch performed a cadaveric experiment
investigating the effect of cutting the ALL and
ITB at 30° of flexion [28]. They used a custom
robot replicating the clinical internal/external
rotation or dial test, while tracking the free
floating tibia in six degrees of freedom [29,
30]. They found division of the ALL in the
ACL intact knee increased internal rotation at
30° of knee flexion by 2.4° (fig. 1). However,
there was wide variation in the effect of ALL
sectioning between specimens, which in one
specimen was not significant but in another
caused an increase in internal rotation
approaching 40% (fig. 2). Sonnery-Cottet, in a
navigation based study, demonstrated increased
internal rotation after division of the ALL in the
ACL deficient knee at 20° and 90°, as well as
increased coupled axial rotation during the
pivot shift [31]. Again using navigation,
Spencer demonstrated an increase in internal
rotation during a simulated early stage pivot
shift after division of the ALL in an ACL
deficient knee [32]. Parsons, using a six degree
of freedom robotic system, found the ALL to
be the primary restraint to internal rotation at
knee flexion angles greater than 35°, with the
ACL providing the greatest restraint closer to
extension [5]. Of note, the ITB was removed
from all specimens in this study prior to testing.
Consistent with this finding, Lording and
Getgood, in a navigation based study with
manually applied forces, found the ALL to play
a significant role in internal rotational control
only at knee flexion angles greater than 30°
[33] (fig. 3).
Fig. 1:
Effect of cutting the ALL on tibial rotation.
Ext rot, External rotation; Int Rot, Internal rotation.