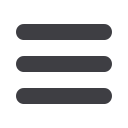
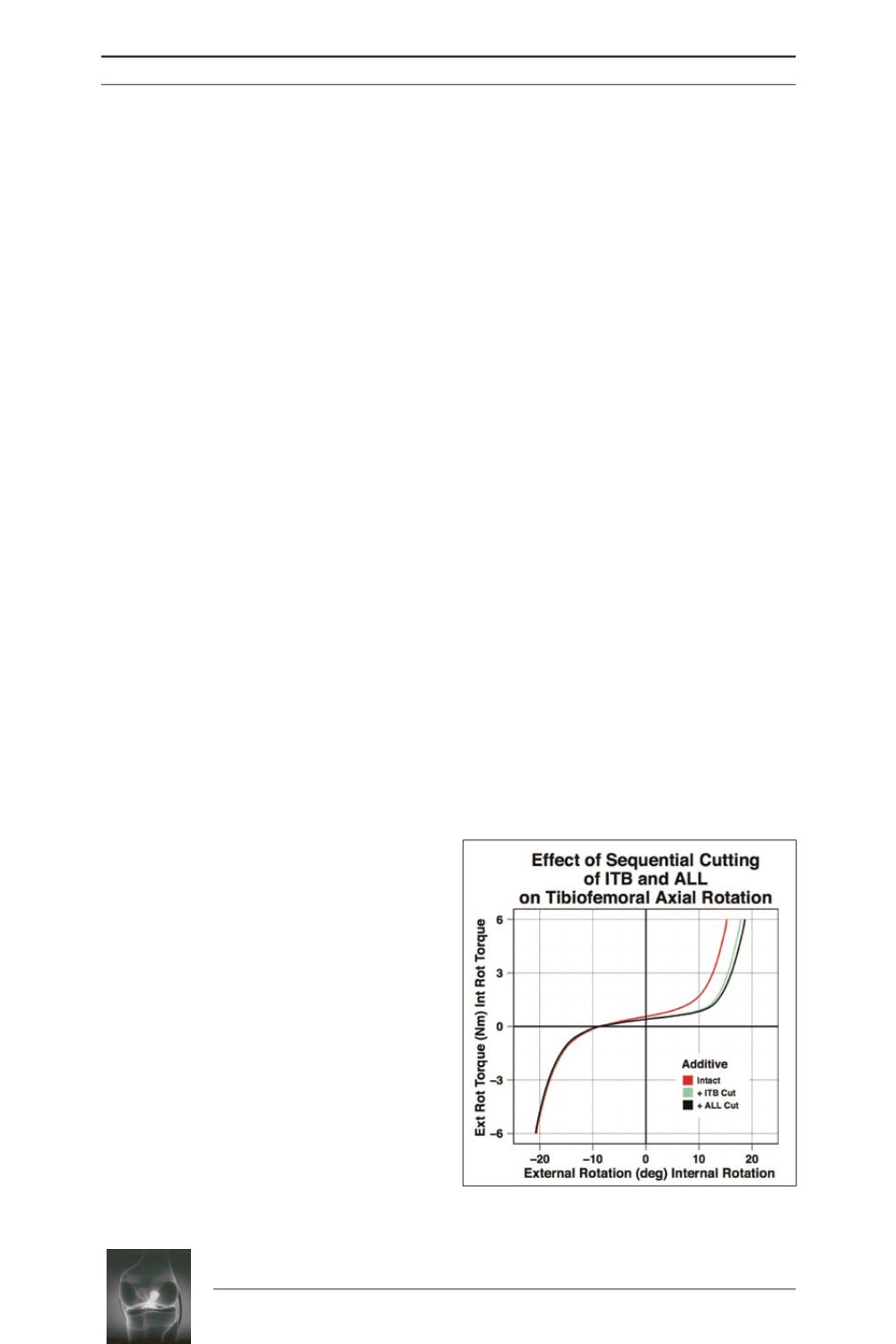
T. LORDING, A. GETGOOD, T.P. BRANCH
34
THE ILIOTIBIAL BAND
The idea that the iliotibial band contributes
to rotational control of the knee is not new.
In fact, the term “anterolateral ligament”
was probably first used by Kaplan in his
1958 morphological study of the iliotibial tract
[35], and was subsequently used by Terry in
1986 to describe the function of the deep and
capsulo-osseous layers thereof [13]. In 1979,
Fetto was able to induce a pivot shift in an ACL
intact knee after division of the ITB [36]. Jakob
noted increased internal rotation but a para
doxical decrease in the pivot shift after release
of the ITB from its distal femoral attachment,
reflecting the complex and multifactorial
nature of the pivot shift phenomenon [37].
When he released the ITB distally by osteotomy
of Gerdy’s tubercle, rotational subluxation
during the pivot shift manoeuver became so
marked in theACLdeficient knee that reduction
did not occur before 60° of flexion.
Anatomically, the insertion of the ITB onto the
lateral distal femur, known as Kaplan’s fibres,
has been shown to be a true tendon enthesis
[38]. This could be considered to divide the ITB
into a proximal, tendinous part, and a distal,
ligamentous part that could contribute to the
control of internal rotation. Terry’s anatomical
study describes the capsulo-osseous layer as
inserting behind Gerdy’s tubercle and identifies
it as the “fibrous pearly band” attached to
Ségond’s eponymous fracture [13, 39].
A number of recent biomechanical studies
support a role for the ITB in the control of
internal rotation. Gadikota, in a robotic study
investigating the effect of increasing ITB load,
found that internal rotation was significantly
reduced between 20° and 30° of knee flexion
with an ITB load of 50 N, and from 15° to 30°
with a load of 100 N, suggesting a dynamic
function [40]. Lording et al measured an
increase in internal rotation of 2.6° in the ACL
intact knee at 30° after division of the ITB at
Gerdy’s tubercle [28] (fig. 4). This increase
was similar in magnitude to that noted after
division of the ALL (2.4°) but with less
variability between specimens. Butler
investigated the impact of ITB division and
tenodesis after single- and double-bundle ACL
reconstructions in cadaveric knees using a
navigation system [41]. The deep layers of the
ITB were released from the femur. Under a
coupled anterior translational force and internal
rotational torque at 30° of knee flexion, internal
rotation increased by 3.9° in the single-bundle
group and by 2.9° in the double-bundle group.
Under pure rotational torque, internal rotation
increased in the single-bundle group at 30° of
flexion by 4.4° and in the double-bundle group
at 90° of flexion by 3.4°. ITB tenodesis using
the superficial ITB reduced internal rotation
compared to the reconstructed knee in all tests.
Kittl found the superficial and deep layers of
the ITB to be the most important stabilizers of
internal rotation, with the superficial layer
more important at deeper flexion angles and the
deep layer especially important in extension
and at 30° of flexion in the ACL deficient knee
[6]. In his study, the superficial and deep layers
of the ITB were also the primary restraints to
the pivot shift. In Sonnery-Cottet’s study,
division of the ITB in the ACL intact knee
caused a significant increase in internal rotation
at 20° of flexion and of coupled axial rotation
during the pivot shift [31]. Sectioning of the
ITB after the ACL and ALL also caused
increased internal rotation at 20°, 90° and
during the pivot shift.
Fig. 4:
Sequential cutting of the ITB and ALL
increased internal rotation by 2.6° and 3.4°.